The Milky Way Laboratory
Decades of research have uncovered the basic formation mechanisms of stars in our own solar neighborhood, however, most stars in the Universe were formed in ‘extreme’ environments with conditions vastly different than the relative calm of our local universe. My research capitalizes on the one cosmologically representative region that is close enough to study individual protostellar cores, the Central Molecular Zone (CMZ, the inner 500 pc of the Milky Way). At UConn, I have founded the Milky Way Laboratory, a research group focused on using our Galaxy as a laboratory for understanding star formation throughout the Universe with both observations and simulations.
Our UConn Astrophysics group is fun-loving, lively, and growing all the time. I hope to hire 1 incoming PhD students in Fall 2023, so please apply if you’re interested and let me know if you have any questions!
Star Formation in the Central Molecular Zone
We have performed the first complete, unbiased, high-resolution survey of the dense star-forming gas in the Central Molecular Zone (CMZ: inner 500 pc of the Milky Way), CMZoom (Battersby et al. 2020) on the Submillimeter Array in Hawai’i. We have developed a catalog of sources in the CMZ that is 99% complete (Hatchfield et al. 2020) and are using this as a finder chart for Atacama Large Millimeter/Submillimeter Array (ALMA) follow-up observations (e.g. Lu et al. 2020, Barnes et al. 2019). CMZoom data is publicly available on the Harvard Dataverse. (Figure from Battersby et al. 2020, shows CMZoom in red, Herschel 70 micron in green, and Spitzer 8 micron in blue.)
Galactic Center Simulations
In the Milky Way Laboratory our approach is manifold. The complex interplay of physical processes involved precludes a simple predictive theory for star formation and necessitates direct comparisons between observations and simulations. We have developed a series of AREPO simulations of our Galaxy’s Center that accurately reproduce its major kinematic and structural features. These simulations include an accurate gravitational potential and star formation physics, including self-gravity and feedback (Sormani et al. 2020, Tress et al. 2020). We have included tracer particles to uncover the origin of CMZ clouds and total Galactic Center inflow rate (Hatchfield et al. 2021). In the next phase, we hope to run high-resolution zoom simulations toward clouds in the simulated CMZ, from which we will construct synthetic observations for direct comparison with observational data. (Figure shows total column density of gas from Sormani et al. 2020.)
3-D CMZ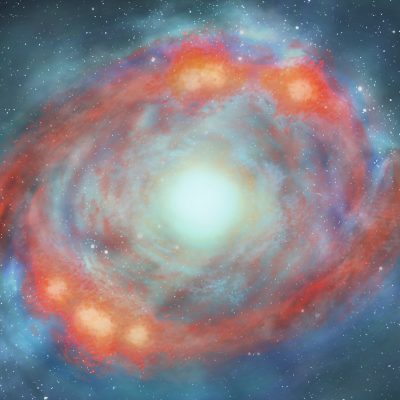
The CMZ is the only galaxy center we can presently study in exquisite detail, yet the diverse physics in this region connects communities across the electromagnetic spectrum. With its extreme properties, the CMZ bridges the gap from our local, quiescent environment to more distant galaxies. The 3-D CMZ project will produce, for the first time, a comprehensive, self-consistent 3-D picture of the CMZ. This map is relevant for communities across astrophysics, impacting constraints on dark-matter annihilation in the Galactic Center and allowing us to test the universality of a star formation-dense gas relationship. We will create a self-consistent, probabilistic 3-D picture of the Central Molecular Zone, (CMZ: the inner 500 pc of the Milky Way), for the first time, including the best available constraints and uncertainties. This map will be physically motivated and consistent with all observational constraints. Image on the right is an artist’s rendition (credit: Francesca Holland) of a top-down view of the two-spiral arm model of our Galaxy’s CMZ, inspired by Henshaw et al. 2016, based on e.g. Sofue et al. 1995, Sawada et al. 2004).
ALMAGAL
ALMAGAL is a Cycle 7 large program on ALMA (2019.1.00195.L, PIs: Cara Battersby, Paul Ho, Sergio Molinari, Peter Schilke) dedicated to studying the evolution of high-mass protocluster formation in the Galaxy. ALMAGAL will observe at 1mm continuum and lines more than 1000 dense clumps with M>500 M_sun and d < 7.5 kpc with similar linear resolution. The sample covers all evolutionary stages from IRDC to HII regions from the tip of the Bar to the outskirts of the Galaxy. The setup with 0.1 mJy sensitivity will enable a complete study of the clump-to-core fragmentation process down to at least 1000 AU and 0.3 M_sun Galaxy-wide, mapping the temperature and the local/global infall velocity patterns of the cores-hosting clumps. ALMAGAL publicly accessible data cubes and catalogs will be an invaluable legacy of ALMA, that will allow numerous community followup studies.
ACES
ACES (ALMA CMZ Exploration Survey) is a Cycle 8 ALMA large program (2021.1.00172.L, PI: Steve Longmore, Co-PIs: John Bally, Cara Battersby, Adam Ginsburg, Jonny Henshaw, Paul Ho, Izaskun Jimenez-Serra, Diederik Kruijssen, Betsy Mills). ACES will derive the gas properties from cloud scales down to the size scale of individual forming stars across the inner 100pc of the Galaxy. This will address fundamental open questions in star formation and galaxy formation/evolution, providing a new benchmark for understanding how the mass flows and energy cycles shape the center of galaxies, regulate star formation and control the activity of the central supermassive black holes.
3-D MC: Mapping Circumnuclear Molecular Clouds from X-ray to Radio
We are combining archival Chandra X-ray data, Herschel data, and ground-based radio data to perform the first 3-D mapping of molecular clouds within the Central Molecular Zone (CMZ) of our Galaxy. Chandra has detected X-ray reflections in the CMZ caused by previous flaring events of SgrA* propagating outwards and interacting with the surrounding molecular gas. As the X-rays propagate radially outwards, they reflect and illuminate different parts of the cloud, mapping it over time, like an X-ray scan. Different epoch Chandra observations slice through the 3-D structure of the molecular clouds. We combine these multi-epoch Chandra X-ray data with archival Herschel and ground-based radio spectral line data to map the molecular clouds in 3-D.
PRIMA (PRobe far-Infrared Mission for Astrophysics) is a Far-IR probe mission concept under development for submission to NASA. Read more about PRIMA’s specifications and science goals on the PRIMA website. The PRIMA concept is of a cryogenically-cooled far-IR observatory, with a sensitive wideband spectrometer and a multi-band spectrophotometric imager / polarimeter. PRIMA will offer the science community the capability to explore a wide range of problems in astronomy, from the growth of stars and black holes over cosmic time, the rise of metals, the role of cosmic magnetic fields, star and planet formation, and the origins of Earth’s water.
Former Projects:
I was a member of the NASA’s Science and Technological Definition Team for the Far-IR Surveyor Mission Concept for the 2020 Decadal Survey. Together with a community of scientists and engineers, we developed the scientifically-compelling and technically-feasible mission concept The Origins Space Telescope. Origins offers a factor of 1000 (yep, you read that right!) improvement in sensitivity over previous observations at these wavelengths, and if selected, Origins will open unprecedented discovery space in the infrared, unveiling our cosmic origins.
Origins will address some of our most compelling questions:
- “How did we get here?
- How do the conditions for habitability develop during the process of planet formation”,
- “How does the Universe Work?:
- How do galaxies form stars, make metals, and grow their central supermassive black holes from reionization to today?”, and
- “Are we alone?:
- Do planets orbiting M-dwarf stars support life?”
To learn more, check out beautiful (and very comprehensive) 376 page final report, available here or read a short summary by yours truly in Nature Astronomy: Battersby et al. (2018).
Milky Way Bones
Despite decades of research, the structure of our Milky Way, such as the number and orientation of spiral arms, remains a topic of much debate. Goodman et al. (2014) argue that very thin, very long Infrared Dark Clouds (IRDCs) may trace out the densest portion of the spiral structure of the Milky Way, a much denser version of the dark dust lanes seen in nearby face-on spiral galaxies. Identifying and characterizing these “Bones of the Milky Way” may ultimately help assemble a global fit to the Galaxy’s spiral arm by piecing together individual skeletal features. We have undertaken a search for more “Bones of the Milky Way” (Zucker, Battersby, & Goodman, 2015 and Zucker, Battersby, & Goodman 2018) and are working to understand their properties. (Figure showing the position-position-velocity structure of Filament 5 in C18O from the IRAM telescope, Battersby et al., in prep.)
High-Mass Star and Cluster Formation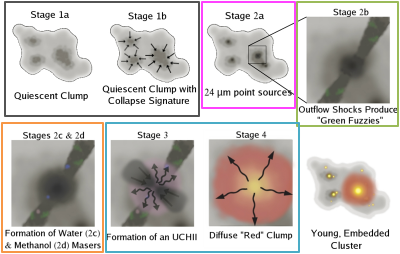
Understanding formation of high-mass stars is complicated by their relative rarity (and therefore higher than average distance), their dusty, highly-extincted birthplaces, and the fact they quickly disrupt their natal environment with their immense feedback. Cara Battersby studied the properties, evolution, and kinematics of high-mass star and cluster formation as part of her PhD thesis (Battersby et al. 2010, 2011, 2014a, 2014b, 2017) and as part of the Bolocam Galactic Plane Survey (Aguirre et al. 2011, Rosolowsky et al. 2010) and Hi-GAL (Molinari et al. 2010, 2011) teams. The Milky Way Laboratory continues to investigate these mysteries, in particular the nature of the most quiescent clumps (Svoboda et al. 2016, 2019), the nature of massive protoclusters (W51, Machado et al., in prep.), and the high-resolution perspective with ALMAGAL. (Figure from Battersby et al. 2010.)